|
|
|
|
|
|
|
|
 |
|
Real Engineering
The applied research poured into the Monaco system is the result of collaborative teamwork, between three friends who worked together at Swift Engineering. Alvin Lloyd, founder of Grand Prix Audio, was crew chief and team manager for several race teams, from S.C.C.A. Formula Ford to IMSA. He then worked at Swift for 13 years. He oversaw the construction of hundreds of race cars, was the primary technical contact for racing teams around the world and coordinated the construction of the earlier mentioned wind tunnel and Indy C.A.R.T. project. For his last two years at Swift, he grew into Vice President of Operations and oversaw all aspects of its operations.
At Swift and during the same 007.i C.A.R.T. project, Henry Wolf worked in the engineering department and was responsible for the engine installations. He later conducted shaker dyno research determining natural resonance frequencies and the stiffness moduli of all aspects of cars and their tires. He's also fluent in program development to test material stress and strain behavior, F.E.A. analysis and the design of hypersonic jet engines at Cal Tech. Alvin and Henry jointly developed the "True Vector" coupling system of the Monaco, which refers to the pin/bearing interfaces between the individual support columns.
Tom Huschilt's expertise includes suspension design and associated deflection/resonance research -- for cars of drivers like Nigel Mansell, Mario and Michael Andretti -- as well as designing his own audio equipment together with his dad, a retired electric engineering professor. During the formative research & development phases of Grand Prix Audio, Tom contributed extensive instrumentation and data acquisition, i.e. conducted a comprehensive survey of existing resonance control solutions while subjecting them to stringent testing and measurements to determine Grand Prix Audio's optimal address for these same problems.
|
|
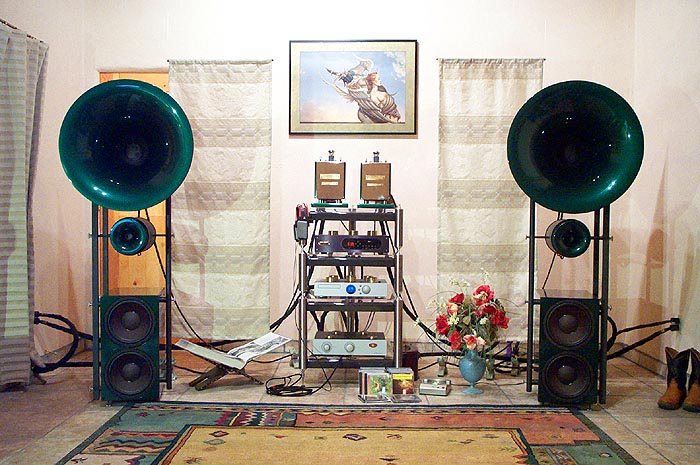 |
|
A brief exchange with Alvin elicited the following panorama.
|
|
 |
|
|
|
|
|
|
Alvin, you're undoubtedly keenly aware of the cesspool that faces the well-meaning but ultimately confused music lover and audiophile while sifting through the maze of conflicting claims and approaches in your chosen field - resonance control in audio components. Could you give us your take on this perplexing situation?
|
|
 |
|
|
|
|
|
|
(Laughs) Cesspool? Is that like being up to your eyeballs in alligators? (Chuckles again.) That's one way of putting it. Let's address a few items we've looked into deeply, to ascertain their relative effectiveness in taming resonance problems in our audio frequency band.
For illustrative purposes, juxtapose self-generated resonances (transformers, CD drives) against floor-borne vibrations (the secondary and temporary ad hoc elements of passing traffic, washing machines, dryers and slamming doors in apartment complexes; and the primary constant impact hammering of your loudspeakers acting as floor-coupled exciter motors) and airborne vibrations (also as generated by your loudspeakers reproducing 30 cycle bass waves at 90dB sound pressure levels). Which ones do you think have the most pronounced impact on your music signal?
|
|
 |
|
|
|
|
|
|
This sounds like a trick question. I'm sure you're about to tell me that self-generated system resonance is merely the tip of ye olde iceberg when compared to the constant high-pressure jackhammering that listening to music entails as a matter of course. Especially with fullrange speaker systems at high output.
|
|
 |
|
|
|
|
|
|
Grasping that wasn't rocket science, was it? Not surprisingly, measurements clearly bear this out. When you're talking resonance control, you're concerned about two things: Frequency and attenuation. What frequencies need to be addressed? How much resonance amplitude does your address subtract from the event? That's it, pure and simple.
The various cones, footers and isolation bearings all do work, but only to a very limited and somewhat erratic extent. They're not addressing the bigger picture. They're ancillary, secondary or tertiary devices.
While multiple axis bearings are being used in the manufacture of silicon wafer computer chips and electron microscope applications, for example, people tend to forget something very important. The rooms that such manufacture or research is conducted in are completely decoupled from their support structure. The building itself conforms to minimum seismic specs. That entails super-thick special concrete foundations with pilings that penetrate deeply into the ground like bridge supports extend far into the ocean or river bed. Try working with a bearing-decoupled electron microscope in an ordinary residence that vibrates each time a truck passes.
|
|
 |
|
|
|
|
|
|
I do contemplate my navel at times, Alvin. Alas, never with a microscope. It ain't that pretty. But I do get your point. Clearly. I'm now about to suspect that the mass-loading approach too requires more than is apparent to the eye - er, scale.
|
|
|
 |
|
|
|
|
|
|
Indeed. Taken to the extreme, it does work reasonably well - but not without damping and multiple degrees of freedom. Envision the following. A mechanic sets up a heavy equipment garage with a solid 6" concrete slab foundation. He installs various gear weighing tons each. Powering up massive motors, saws, hydraulics, pumps, routers or what have you still affects the different workstations. Our mechanic will have to experiment with precise placement to minimize exposure to these standing waves. Furthermore, he has to install elastomeric isolation dampers underneath his equipment to minimize the interference these machines exert upon each other by propagating their self-resonance through the concrete. And this with multiple tons of weight, not a few hundred pounds of lead shot inside a component stand. As an aside, there isn't an industrial scenario extant that would couple high mass to hard metal footers. That simply doesn't work. What's required are multiple degrees of freedom to attenuate vibration.
Have you ever listened to your system barefoot? Played some bass-heavy music perhaps? Noted at times how certain notes set off the whole floor? The energy tickles your feet and moves up your spine?
|
|
 |
|
|
|
|
|
|
Bass-heavy music? Like Crap you mean? What do you take me for, AIvin? I'm an audiophile. I listen to string quartets exclusively. I have no idea what you're talking about.
|
|
|
 |
|
|
|
|
|
|
That's what I thought. Too bad. Busted, eh? (Chuckles.) So you see, in terms of effectiveness -- how much effort is required for how much return -- the high-mass approach of extreme rigidity performs quite poorly because you can never apply enough mass. It would work theoretically if you just could bring enough sheer weight and proper material density to bear. But remember, even a few tons won't do the trick.
For Grand Prix Audio, we thus decided on a multi-pronged approach. We exploit space-age composite materials with superior self-damping properties. We mate those with the design's intrinsic six degrees of freedom of motion that acts like a shock absorber. But a shock absorber without damping -- if you've ever seen a car go down the road with one shock shot -- is not a pretty sight. So we add effective decoupling and damping interfaces. They minimize resonance transfer. Then we optimize the effectiveness of the acrylic and Sorbothane materials by tuning them according to the exact weight supported.
To illustrate the multi-axis freedom of motion, gently manipulate a single shelf atop its three Sorbothane washers while holding the uprights steady. You can modestly displace the shelf horizontally by 360 degrees. Additionally, you can compress the Sorbothane footers in the vertical axis. The inherent rebound is a simple function of tailoring their thickness and composition to the weight supported.
Now add the horizontal range of motion inherent in each True Vector coupling, of which there are two stages - between shelves 2/3 and 3/4 -- and the give of the viscous interfaces between columns and crossmembers. When you compound these multiple dimensions of range of motion, you arrive at 6 degrees of freedom, the proper standard of resonance control in all industries.
In toto, the Monaco structure incorporates eight discreet stages of resonance control. Those work in concert to maximize their combined effectiveness. It's the old maxim of encircling the camp before the attack rather than attempt to break through the front gate by brute force. That only results in a focused counter-defense from the camp's inhabitants - they'll push back at you with a vengeance.
|
|
|
|
|
|
Alvin's warpath example coupled with the preceding illustrations made perfect sense to my non-racing, non-engineering brain. But then so have other explanations, from other designers who came to quite different conclusions in support of their particular solutions.
Whom do you believe? We all intuit that not every good story is true. Not every white paper is truly lily-white. More often than not, it's thinly disguised marketing propaganda, pickled with easy-grasp arguments that are flawed because they are only factual when viewed in isolation. Just think of the mechanical diode nonsense that magically drains resonance out of components as though it was a one-way valve. The clever parallel between resonance and a water downspout seems to make sense. It's visual. Hence we buy into it to spout popular wisdom, without grasping that we've really been hoodwinked by a talented marketing guy. (Having been such a spin doctor, I'm allowed to say that.)
As always, the proof of the stew is whether you get the flu (or should that be flew). How did the Monaco alter the sound in my system? Did it introduce any negatives? One thing was clear from the word go - no break-in. Halleluja! No placement agonizing. No twiddling with toe-in, path lengths, early reflections, optimal listening distance. None of the usual neurotic audiophile crap that, nonetheless, is important to address. No, for once, this was going to be plunk down squared: Park the stand and my butt, be off chasing rainbows and counting violins. Instant gratification. How American!
Ready?
|
|
 |
|
|
|
 |
 |
|
|
 |